[ad_1]
Poultry waste is one of the utmost imperative pollutants if not correctly disposed of. To increase the nutritional value of poultry feather wastes that can be utilized as animal feed, it is possible to chemically or biologically treatment of chicken feathers. If correctly handled to minimize negative consequences, poultry waste can be effectively used to create a variety of value-added products, such as fertilizer, biofuel, and animal feed3.
The obtained data from the structured questionnaire during the survey
The main poultry operations are widely distributed in the investigated areas (deep litter and battery cage system). The number of birds reared in a deep litter system was exceeded by 20,000 birds/cycle compared to a battery cage system of 4000 birds/cycle. Twenty-eight deep litter farms (70.0%) reported having shed locked in order to segregate and/or isolate their premises for disease control, whereas five battery cage systems (50.0%) only have a locked gate around the building as shown in Table 1. Maduka et al.25 exhibited that the main components of biosecurity practices included fences around buildings, gates, and all in all out management represented about 80–90%. Furthermore, Mustafa26 recorded that the primary outlines of protection against any disease transmission are a closed gate and fence around it. Besides that, fence was not available for most farms in both semi-modern and conventional systems.
Furthermore, at the entry gate of the building, application of footbath dip is presented at 70% in the battery cage system compared to 23 out of 40 (57.5%) in the poultry farms of the deep litter system in this study. Haftom et al.27, found that at the gate, footbaths were used by 80% of the broiler poultry farms, while 88% of the farms practiced washing and disinfecting their buildings and equipment. Ali et al.28 indicated that a high level of biosecurity was applied in the closed system than in the open system, whereas 84.6% was used in the footbath dip at the entrance of the shed. On the contrary, the isolation rate of sick birds in separated areas was similarly high in both investigated systems, at 77.5 and 80%, respectively, to avoid dissemination of highly pathogenic diseases. Sudarnika et al.29 found that twenty-four poultry farmers segregated sick birds from healthy birds at 96% and disposed of them by burning or burying them. Meanwhile, just two poultry farms left dead birds thrown away at 4.4%. Furthermore, Mohammed and Helal30 found that most respondents pointed out that they isolated the diseased chicks in a chosen area by using the same building as the rest of the flock. In addition, hygiene stations were rarely present in some poultry premises, and the absence of biosecurity plans employed on-farm was concerning.
In a deep litter system, the litter types that used were sawdust and wood shavings at 37.5 and 62.5%, respectively. For litter disposal during the cycle, some broiler poultry farms clear all litter (15.0%), while 85.0% of these farms remove 10 cm from the top layer and add another. On the other hand, in a battery cage, manure is disposed of during cycle 100% in a tray that is far away from the birds and then collected in manure areas. The frequency of litter change in deep litter was 67.5% once/week while other farms were at 32.5% every month. The methods of disposal of poultry mortalities were incineration followed by disposal in landfill and burial, especially in deep litter (40.0, 25.0 and 20.0%), while in battery cages, incineration and burial were the most applied methods of mortality disposal (50.0 and 30.0%, respectively). Mohammed and Helal30 stated that participants in each poultry operation system clarified lack of capital and sufficient space for applying hygienic measures of disposal of dead birds that involve burning or burial. Besides, poultry producers did not apply composting as a safe method to dispose of dead birds. Furthermore, the risk of environmental degradation and disease transmission is increased when poultry carcasses are dumped in waterways or on a road where dogs might find them and scavenge. Moreover, Muduli et al.3 reported that strict monitoring of the burial of dead birds and/or mortalities on the farm is required to avoid contamination of groundwater sources; additionally, composting could be used to reduce bacterial pathogens and then recycled as soil fertilizer. In the current text, mortalities disposed in waterways were 20% in battery cages as compared to 15.0% in deep litter systems. Disinfection in between cycles was available in both systems, whereas 77.5% in the deep litter system and 70% of the battery cage applied. Finally, the poultry producers reported that the mortality rate/cycle was significantly greater in deep litter (12.0%) than in battery cage (10%) at P 0.005 as presented in (Table 1). Turkson and Okike31 mentioned that to prevent and control highly pathogenic diseases such as HPAI H5N1, the application of biosecurity measures is a critical point. Additionally, the majority of small-scale broiler chicken farms employ minimal or no biosecurity controls, which may raise the likelihood of disease transmission between poultry farms, mortality rates, and the danger of exposing people to potential health risks32.
The distribution pattern of pathogenic bacteria from liquid and solid waste
The frequent distribution of pathogenic microbes arising from investigated farms in Table 2 clarified that 87.62% (184/210) of the total examined samples positively contained highly pathogenic bacteria. The most predominant bacterial isolates from waste were E. coli (33.69%, 62/184), Salmonella spp. (26.09%, 48/184), followed by K. pneumonae (15.22%, 28/184), and L. monocytogenes (14.13%, 26/184). Meanwhile, Shigella flexneri was detected in the least percentage (10.87%, 20/184). The highest percentage of E. coli was isolated from chicks dropping (46.43%, 13/28), manure collected area (40%, 12/30), and wastewater (37.04%, 10/27) followed by mortalities collected area (32.14%, 9/28) and chicks’ litter (30%, 9/30). Oppositely, Salmonella spp. was recorded in the highest percentage in wastewater (37.04, 10/27) and chicks dropping (28.57%, 8/28) followed by mortalities collected area (25%, 7/28) whilst K. pneumonae was isolated at a higher rate from mortalities collected area (21.43%, 6/28) followed by both chicks’ litter and manure collected area (16.67%, 5/30 each). Furthermore, L. monocytogenes was highly isolated from waste feed (27.78%, 5/18) and feathers (17.39%, 4/23). Besides, Shigella flexneri was also detected in feathers (21.74%, 5/23) and chicks’ litter (13.33%, 4/30). These findings support those of Sahoo et al.33 who showed that managing poultry litter had a significant impact on the health of birds. Keeping the chicks’ litter dry is another essential aspect of managing chicken farms. In the presence of elevated litter pH and moisture content, Soliman et al.34 explained that chicks’ litter is a favorable medium for bacterial growth and transmissions like S. Typhimurium. Additionally, Tiweri et al.35 noted that L. monocytogenes was frequently found in the vicinity of animals and persisted for an extended period of time in animal waste, soil, water, and feed. According to Abdel-Latef and Mohammed36, contamination of the poultry environment by highly pathogenic bacteria is the main reason for greater death rates and large economic losses in these farms. Environmental contamination may be caused by bird fecal droppings reflecting less stringent hygiene practices in poultry farms.
The total bacterial count and indicator microorganisms isolated from liquid and solid waste
The total viable count and indicator bacteria that were identified from liquid and solid waste that the deep litter system produced were displayed in Table 3. It was discovered that the TVCs in both mortalities and manure collected areas were significantly greater (8.21 × 107 ± 1.2 × 105 and 7.32 × 107 ± 2.3 × 10 CFU/100gm) followed by chicks’ litter (6.71 × 107 ± 3.5 × 10 CFU/gm) and wastewater (3.56 × 107 ± 1.1 × 105 CFU/mL) compared with its count in feathers and waste feed (2.34 × 104 ± 1.1 × 10 and 2.34 × 105 ± 1.1 × 105 CFU/gm, respectively). In addition, TCCs were isolated at the highest rates in chicks’ litter and manure collected areas (900 ± 1.1 and 900.0 ± 4.8 CFU/100gm), whilst in waste feed it was 110.0 ± 6.2 CFU/100gm. As well, FCCs were significantly high in both chicks’ litter and manure collected areas (350.0 ± 4.1 and 350.0 ± 3.0 CFU/100gm, respectively) followed by chicks dropping (220.0 ± 1.2 CFU/100 gm) and wastewater (220.0 ± 2.2 CFU/100 mL). Meanwhile, FCCs in feathers and waste feed did not exceed 60.0 ± 3.6 and 90.0 ± 1.1 CFU/100 gm, respectively. Abd El-Salam et al.37 found that the wastewater contains 1600 colonies of total coliform. Hartel et al.38 pointed out that the possible source of fecal coliforms is fresh poultry litter, and the composting process of litter can principally eradicate these bacteria. Nevers et al.39 clarified that fecal contaminations including livestock, poultry, and other fecal wastes are potential sources of bacterial pathogens with human health risks in recreational waters. Zhuang et al.40 noted that chicken farms are a crucial source of fecal pollution in the environment as poultry excrement contains bacteria that are harmful to the environment and humans.
Characterization of Ag NPs and Ca(OCl)2-Ag NPs using TEM and FT-IR
TEM of Ag NPs showed the morphological shape (spherical and elliptical) and the size of nano-silver particles ranged between 19.07–34.47 nm (Fig. 1a,b). TEM photography of Ca(OCl)2-AgNPs revealed the spherical and elongated morphological shape of the composite’s nanoparticles (NPs). Besides, the diameter of the NPs ranged from 4.94 to 33.62 nm (Fig. 2a,b). Ag NPs (Fig. 3a) showed specific peaks at 3272.18, 1638.07, 919.01 and 604.61 cm−1. Furthermore, FT-IR of Ca(OCl)2-AgNPs (Fig. 3b) showed characteristic peaks at 3273.57, 2132.25, 1638.21 and 602.51 cm−1, confirming the successful loading of Ca(OCl)2 on the Ag NPs. Roy et al.41 pointed out that the FT-IR spectra of nano-silver particles exhibited the characteristic peak of Ag NPs that is located at 1638 cm−1. In addition, Mohammed16 displayed FT-IR of Ca(OCl)2 loaded on Ag NPs whereas a specific peak appeared at 2480 cm−1, approving the loading in a successive way.
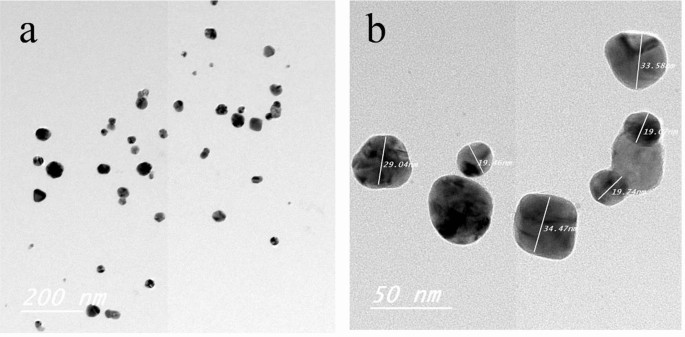
TEM photography of nano-silver particles (Ag NPs). The morphological shape (a) showed the fine spherical and elliptical particles of nano-silver besides the diameter of NPs (b) was ranged from 19.07- 34.47 nm.
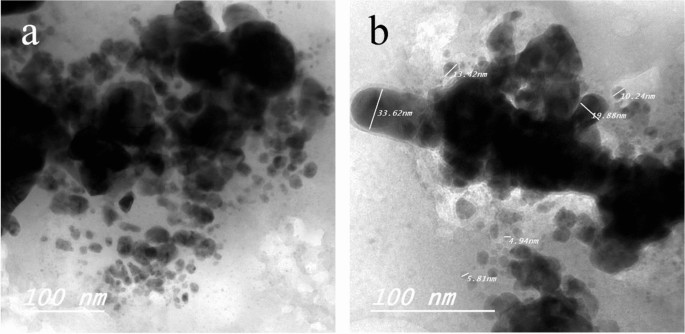
TEM photography of Ca(OCl)2 loaded on Ag NPs (a–b). The morphological shape displayed the spherical and elongated nanoparticles (NPs) of composite (a). Besides, the diameter of the NPs (b) ranged from 4.94 to 33.62 nm.
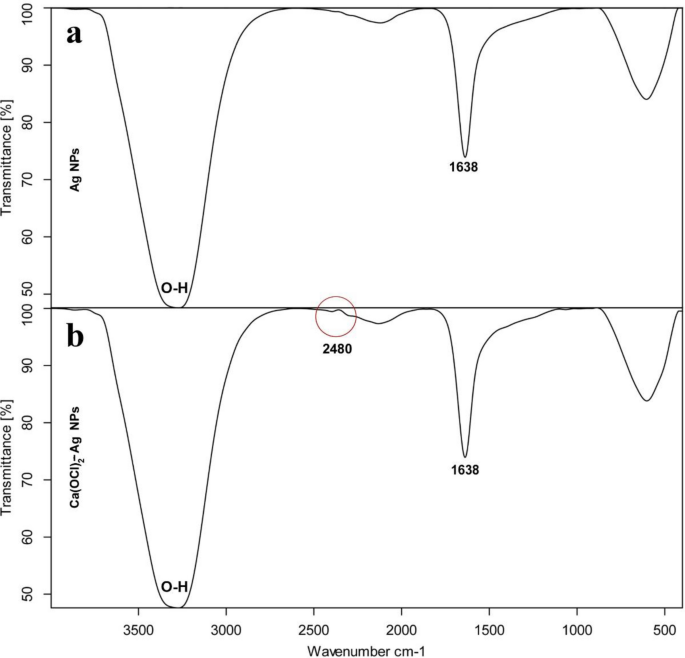
FT-IR spectrum of Ag NPs (a) and Ca(OCl)2-AgNPs (b).
The antimicrobial activity of disinfection compounds, Ag NPs and Ca(OCl)2-Ag NPs
Pathogenic bacteria were isolated from several waste types and their susceptibility to the disinfection products, Ag NPs and Ca(OCl)2-Ag NPs (Table 4) revealed that the susceptibility of all isolated bacteria to VIRKON S was not greater than 70% at the highest concentration of 2% after 24 h of exposure when compared to the lowest concentration, whereas their susceptibility was between 30–60%. As well, the susceptibility of isolates to quaternary ammonium compounds was not exceeded by 80%, except that L. monocytogene was highly sensitive at 100% at a concentration of 1.5 mg/L. Møretrø et al.42 clarified that due to the presence of resistance genes, L. monocytogenes was tolerant to sub-lethal concentrations of QAC.
Ortiz et al.43 clarified that there is a positive association between frequent use of a QAC disinfectant and the existence of L. monocytogene resistant to it which might be attributed to the presence of resistance genes to QAC disinfectants44. In this context, L. monocytogene was significantly more sensitive to Ca(OCl)2 (100%), followed by K. pneumonae, Salmonella spp., and Shigella flexneri, which were 90% sensitive at 1.5 mg/L (P ≤ 0.05). Yim et al.45 discovered that Ca(OCl)2 and QAC were more effective than sodium hypochlorite at completely eliminating vegetative cells and spores. Oppositely, in this study, all bacteria exhibited resistance profiles to Ag NPs that exceeded 30% at concentrations of 5.0 mg/L at 24 h of exposure times compared to the highest concentration of 15 mg/L where the susceptibility of isolates was exceeded 80% for L. monocytogene and k. pneumonae. Furthermore, Shigella flexneri was 100% sensitive. Belluco et al.46 concluded that the overdue effect of Ag NPs on the pathogenic bacteria might have been caused by the slow release of silver ions from the Ag NPs. The effectiveness of Ca(OCl)2-Ag NPs against pathogenic bacterial isolates was investigated in the current study, which found that bacterial isolates (E. coli, K. Pneumonae, Shigella Flexneri, and L. monocytogene) from various waste types were highly sensitive (100%) to Ca(OCl)2-AgNPs at a concentration of 1.0 mg/L after 24 h of exposure. Salmonella spp. were 90% sensitive to Ca(OCl)2-AgNPs at the lowest concentration of 0.5 mg/L.
Silver ions’ ability to bind to Ca(OCl)2, penetrate bacterial cell membranes, and improve membrane permeability may be responsible for this action, confirming that the biocidal activity demonstrated by Ca(OCl)2-Ag NPs is synergistic. These results are consistent with those reported by Morones et al.47 and Sondi and Salopek-Sondi48, who found that employing Ag NPs to treat water increased cell membrane permeability and leakage of the cytoplasm of E. coli. Additionally, Ag NPs have been shown to have an antimicrobial effect is credited with the release of Ag ions from the Ag NPs surface and binding on thiol groups in membrane proteins, resulting in bacterial enzymatic systems are inhibited and DNA aggregation49,50. Mohammed16 found that the microbial effect of Ag NPs against E. coli and S. aureus was exceeded by 80%, whilst it has a lethal effect against K. pneumoniae (100%) at the highest concentration (5.0 mg/L) after exposure time (180 min).This could be due to Ag ions’ ability to bind to and infiltrate the microbial cell membrane. Furthermore, Dilarri et al.51 demonstrated that the Ca(OCl)2 mechanism of action targets the microorganism’s cytoplasmic membrane, which may be responsible for cell death.
[ad_2]